Scientific Revolutions: Fast and Slow
12 March 2016
Saturday

Illustrations from the early scientific revolution bear the stamp of an earlier and other civilization, as in this image, in which as much time has been spent on the trees and the clouds as the scientific experiment itself.
It is a convention of historiography to refer to the formative period of early modern science as “the scientific revolution” (with the definite article), and this is justified in so far as the definitive features of experimental science began to take shape in the period from Copernicus and Galileo to Newton. But in addition to the scientific revolution understood in this sense as a one-time historical process that would not be repeated, there is also the sense of revolutions in science, and there are many such revolutions in science. This sense of a revolution in scientific knowledge has become familiar through the influence of Thomas Kuhn’s book, The Structure of Scientific Revolutions. Kuhn made a now-famous distinction between normal science, which involves the patient elaboration of a scientific research program, and revolutionary science, which involves the shift (a paradigm shift) from an established scientific research program to a new and often unprecedented scientific research program.
Some revolutions in science happen rather rapidly, and some unfold over decades or even centuries. The revolution in earth science represented by geomorphology and plate tectonics was a slow-moving scientific revolution. As long as we have had accurate maps, many have noticed how the coastlines of Africa and South America fit together (a sea captain pointed this out to my maternal grandmother when she was a young girl). When Alfred Wegener put first put forth his theory of plate tectonics in 1912 he had a great deal of evidence demonstrating the geological relationship between the west coast of Africa and the east coast of South America, but he had no mechanism by which to explain the movement of continental plates. The theory was widely dismissed among geologists, but in the second half of the twentieth century more evidence and a plausible mechanism made plate tectonics the central scientific research program in the earth sciences. I have observed elsewhere that Benjamin Franklin anticipated plate tectonics, and he did so for the right reasons, so if we push the origins of the idea of plate tectonics back into the Enlightenment, this is a scientific revolution that unfolded over hundreds of years.
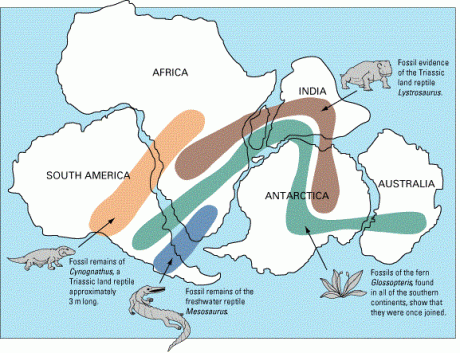
Alfred Wegener recognized fossil patterns over now-separated continents, which suggested a different arrangement of continents in the past, but Wegener had no causal mechanism to explain the movement (map by jmwatsonusgs.gov – United States Geological Survey – http://pubs.usgs.gov/gip/dynamic/continents.htmlen:Image:Snider-Pellegrini_Wegener_fossil_map.gif)
In the past, when knowledge was disseminated much more slowly than it is today, we are not surprised to learn that the full impact of the Copernican revolution unfolded over centuries, while today we expect the dissemination of major scientific paradigm shifts to occur much more rapidly. Indeed, we have the recent example of the discovery of the accelerating expansion of the universe as a perfect instance of a major and unexpected scientific discovery that was disseminated and accepted by most cosmologists within a year or so.

‘The data summarized in the illustration above involve the measurement of the redshifts of the distant supernovae. The observed magnitudes are plotted against the redshift parameter z. Note that there are a number of Type 1a supernovae around z=.6, which with a Hubble constant of 71 km/s/mpc is a distance of about 5 billion light years.’ (quoted from ‘Evidence for an accelerating universe’ at http://hyperphysics.phy-astr.gsu.edu/hbase/astro/univacc.html)
The facility with which the accelerating expansion of the universe was assimilated into contemporary cosmology could be used to argue that this was no revolution in science (or it could be said that it was not a “true” revolution in science, which would suggest an application of the “no true Scotsman” fallacy — what Imre Lakatos called “monster barring” — to scientific revolutions). The discovery of the accelerating expansion of the universe may be understood as an extension of the revolution precipitated by Hubble, who demonstrated by observational astronomy that the universe is expanding. Since Hubble’s discovery of the expansion of the universe it has assumed that the expansion of the universe was slowing down (a rate of deceleration already given the name of the “Hubble constant” even before the value of that constant had been determined). Hubble’s work was rapidly accepted, but its acceptance was the culmination of decades of debate over the size of the universe, including the Shapley–Curtis Debate, so we can treat this as a slow revolution or as a rapid revolution, depending upon the historical perspective we bring to science.

Harlow Shapley (left) and Heber Curtis (right) debated the structure and size of the universe in a famous confrontation in 1920.
While general relatively came to be widely and rapidly adopted by the scientific community after the 1919 eclipse observed by Sir Arthur Eddington, I have noted in Radical Theories, Modest Formulations that Einstein presented general relativity in a fairly conservative form, and even in this conservative form the theory remained radical and difficult to accept, due to ideas such as the curvature of space and time dilation. After the initial acceptance of general relativity as a scientific research program, the subsequent century has seen a slow and gradual unfolding of some of the more radical consequences of general relativity, which became easier to accept once the essential core of the theory had been accepted.

Einstein formulated his field equations for general relativity in 1915, and we are still deducing the consequences of the theory.
It might be hypothesized that radical theories are accepted more rapidly when a crucial experiment fails to falsify the theory, and the more radical consequences of the theory are fudged a bit so that they do not play a role in galvanizing initial resistance to the theory. If Einstein had been talking about black holes and the expansion of the universe in 1915 he probably would have been dismissed as a crackpot. Another way to think about this is that general relativity appeared as a rigorous, mathematically formalized theory with specific predictions that admitted of crucial experiments within the scope of science at that time. But such a fundamental theory as general relativity was bound to continue to revolutionize cosmology as long as later theoreticians could elaborate the theory initially formulated by Einstein.

Jan Hendrik Oort, for whom the Oort Cloud is named, and an early discoverer of the influence of dark matter on cosmology.
This discussion of slow-moving revolutions in cosmology brings us to the slow moving revolution that is coming to a head in our time. The recognition of dark matter, i.e., of something that accounts for the gravitational anomalies brought to attention by observational astronomy, has been slow to unfold over the last several decades. Two Dutch astronomers, Jacobus Kapteyn and Jan Oort (known for the eponymously-named Oort Cloud, suggested the possibility of dark matter in the early part of the twentieth century. Fritz Zwicky may have been the first person to use the term “dark matter” (“dunkle Materie“) in 1933. Further observations confirmed and extended these earlier observations, but it was not until the 1980s that the “missing” dark matter came to be widely recognized as a major unsolved problem in astrophysics. It remains an unsolved problem, with the best guess for its resolution being the theoretically conservative idea of an as-yet unobserved subatomic particle or particles that can be located within the standard model of particle physics with a minimum of disturbance to contemporary scientific theory.
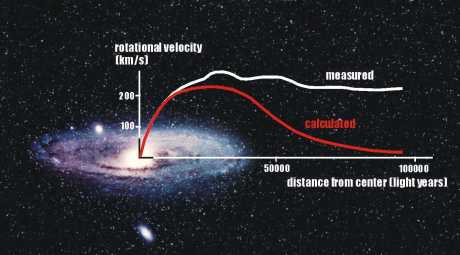
An elegantly simple demonstration of how dark matter shapes the universe: the rotation curve of spiral galaxies cannot be accounted for by the luminous matter in the galaxy.
There are two interesting observations to be made about this brief narrative of dark matter:
1) The idea of dark matter emerged from observational astronomy, and not as a matter of a theoretical innovation. Established theoretical ideas were applied to observations, and these ideas failed to explain the phenomena. The discovery of the expansion of the universe was also a product of observational astronomy, but it was preceded by Einstein’s theoretical work, which was already accepted at that time. Thus a number of diverse elements of scientific thought came together in a scientific research program for cosmology — a program the pursuit of which has revealed the anomaly of dark matter. There is, at present, no widely accepted physical theory that can account for dark matter, so that what we know of dark matter to date is what we know from observational astronomy.
2) No one has a strong desire to shake up the established theoretical framework either for cosmology or for fundamental physics. In other words, a radical theoretical breakthrough would upset the applecart of contemporary science, and this is not a desired outcome. The focus on dark matter as an undiscovered fundamental particle banks on the retention of the standard model in physics. Much as been invested in the standard model, and science would be more than a little out to sea if major changes had to be made to this model, so the hope is that the model can be tweaked and revised without greatly changing it. One approach to such change would be via what Quine called the “web of belief,” according to which we prefer to revise the outer edges of the web, since changing the center of the web ripples outward and changes everything else. The scientific research program at stake — which is practically the whole of big science today, with fundamental physics just as significant to astrophysics as observational astronomy — is an enormous web of belief, and if you got down to a fine-grained account of it, you would probably find that scientists would disagree as to what is the center of the web of belief and what is the periphery.
I suspect that it may be the case that, the more mature science becomes, the more difficult it will be for a major scientific revolution to occur. Any new theory to replace an old theory must not only explain observations that cannot be explained by the old theory, but the new theory must also fully account for all of the experiments and observations explained by the established theory. Quantum theory and general relativity are the best-confirmed theories in the history of physical science, and for any replacement theory to supplant them, it would have to be similarly precise and well confirmed, as well as being more comprehensive. This is a tall order. Early science picked the low-hanging fruit of scientific knowledge; the more we accumulate scientific knowledge, the more difficult it is to obtain more distant and elusive scientific knowledge. Today we have to build enormous and expensive instruments like the LHC in order to obtain new observations, so each round of expansion of scientific knowledge must wait for the newest scientific instrument to come on line, and building such instruments is becoming extremely expensive and can take decades to complete.
Partly in response to this slowing of the discovery of fundamental scientific principles as science matures, we can seen a parallel change in the use of the term “revolutionary” to identify changes in science. It is somewhat predictable that if a new particle is discovered that can account for dark matter observations, this discovery will be called “revolutionary” even if it can be formulated within the overall theoretical context of the standard model, rather than overturning the standard model. In other words, less is required today for a discovery to be perceived as revolutionary, but, at the same time, it is becoming ever more difficult even to achieve this lower standard of revolutionary change in science. It is extremely unlikely that the macroscopic features of the contemporary astrophysical research program will change, even if the standard model were overturned by a discovery related to dark matter. We will continue to use telescopes and colliders to observe the universe and use computers to run through simulations of incredibly complex models of the universe, so that both observational and theoretical astrophysicists will have a job for the foreseeable future.
. . . . .

Perhaps the most studied avenue to augment the standard model to account for dark matter is the supersymmetry (SUSY) approach, which posits a massive shadow particle for every known particle of the standard model.
. . . . .
. . . . .
. . . . .
. . . . .